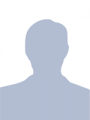
Katja[a]
Okadaic Acid-Induced Apoptosis in Malignant Glioma Cells
from Neurosurgical Focus
Bimal G. Rami, M.D., Lawrence S. Chin, M.D., Barbara E. Lazio, M.D., Satyendra K. Singh, Ph.D.
Abstract and Introduction
Abstract
Object: Okadaic acid (OA), a potent protein phosphatase inhibitor, has been known to induce apoptosis in a variety of cell types. The authors attempted to characterize further this model by identifying proteins involved in this form of programmed cell death.
Methods: Cellular proliferation was assessed using a colorimetric nonradioactive proliferation assay and cell counts. Apoptosis was determined by fluorescent microscopy. Activation of the mitogen-activated protein kinase (MAPK) pathways was determined by immunoprecipitation of extracellular signal-regulated kinase (ERK), c-Jun-N-terminal kinase (JNK), and p38 followed by in vitro kinase assays. Western blot analyses were conducted to show inhibitory-B (IB) phosphorylation and degradation as well as Bax upregulation. The binding of nuclear factor-B (NFB) was shown by electrophoretic mobility shift assay.
Okadaic acid induced cell death in T98G human malignant cell lines (50% inhibiting concentration = 20-25 nM). In T98G cells YO-PRO fluorescent staining was identified, thus indicating an apoptotic mechanism with a smaller percentage of cells undergoing necrotic cell death. Additionally OA induced JNK and MAPK activities in a time-dependent manner, increased the expression of Bax, and increased IB phosphorylation and NFB activation. There was a temporal correlation between these subcellular events and the detection of apoptosis morphology in glioma cells.
Conclusions: The authors believe that OA acts by blocking dephosphorylation events, thus activating apoptotic pathways through ERK and JNK activity. Additionally Bax, IB and NFB may also play a role in regulating these pathways.
Introduction
Apoptosis is a complex process of great importance to normal cell function and development.[6] Deregulated apoptosis has been implicated in a number of pathological disorders resulting in increased cell survival or excessive cell death.[39] Regulation of the intracellular pathways responsible for maintaining the proliferation and apoptotic signals within the cell are poorly understood. Normal and neoplastic cells respond to extracellular stimuli through signal transduction cascades from the plasma membrane to the nucleus.[20] The MAPK family, which includes extracellular signal regulated kinase ERK, p38, and JNK, has been shown to transmit both proliferative and apoptotic signals.[16,24] Induction of JNK and p38 is observed in growth factor-deprived PC-12 cells that undergo apoptosis, an effect that is prevented by transfection of a dominant negative JNK construct.[45] Protein phosphatases have been shown to play an integral role in the regulation of protein kinase signal transduction in multiple human cell lines.[1,28,47] Therefore, we hypothesized that these PPs may also be of importance in apoptotic signal transduction in glioma cells.
The transcription factor NFB contributes to the cellular response to diverse stimuli including cytokines, ionizing ration, and reactive oxygen species.[42] Regulation of NFB occurs through an inhibitory molecule, IB, that binds noncovalently to NFB and prevents its translocation into the nucleus.[2] When the cell senses the appropriate external stimulus, IB is phosphorylated by a recently identified kinase and then degraded, thus releasing NFB to the nucleus.[13] The effect of NFB on apoptosis is unclear; the authors of several recent investigations have shown that it plays a protective role against tumor necrosis factor--induced apoptosis.[3,27,30,40,43]
The culminating stages of apoptosis require the function of proteases that degrade cellular ultrastructure and nucleic acids. The cysteine proteases, interleukin-1-converting enzyme and CPP-32, cleave selectively at aspartate residues and are members of the caspase family of proteins.[6,38] Caspases have recently been shown to activate during chemotherapy-induced apoptosis in tumor cells.[23,33,51] In addition, stable transfection of an inducible interleukin-1-converting enzyme construct into a glioma cell line causes and apoptotic cell death.[50]
The caspases are homologous to the nematode Caenorhabditis elegans death gene ced-3, which may be counteracted by expression of ced-9 and can substitute for its function in C. elegans.[41] Coimmunoprecipitation experiments involving Bcl-2 led to the discovery of Bax, a protein that promotes apoptosis.[11,32,48,49] Excess Bcl-2, however, neutralizes Bax by forming heterodimers, thus resulting in suppression of apoptosis. It has been proposed that the relative intracellular levels of Bax and Bcl-2 function as the cellular "rheostat" that determines whether apoptosis will occur.[31]
Okadaic acid is a membrane-permeable C38 polyether fatty acid that inhibits serine/threonine PP1 and PP2A.[35] Okadaic acid induces apoptosis in several tumor cells but has also been demonstrated to act as a tumor promoter similar to phorbol esters.[14,17,22,29,36,37] Although there is evidence that OA is a potent in vitro and in vivo activator of NFB DNA binding,[34,46] this has never been studied in glioma cells. In this report, we describe OA-induced cell death in malignant glioma cell lines. We focus on the T98G cell line, which has been extensively studied in our laboratory.[7-9] Our data suggest that PPs play a critical role by attenuating the apoptotic pathway signaled by either ERK or JNK activity. We also show that OA causes the activation of NFB and Bax upregulation.
Materials and Methods
Cell Culture
Stock cultures of T98G cells were obtained from American Type Culture Collection (Rockville, MD) and cultured in Eagle minimum essential medium supplemented with both 10% fetal bovine serum (Biofluid, Rockville, MD) and glutamine (2 mM). Cells were grown at 37°C in a humidified incubator with 5% CO2. The proliferative rate for all three cell lines is similar.
Cell Counting Procedures
The number of living cells was assessed using the colorimetric nonradioactive proliferation assay, CellTiter 96 (Promega, Madison, WI), consisting of MTS (Owen reagent; 2 mg/ml) combined with the electron coupling reagent, phenazine methosulphate (0.92 mg/ml). Cells were plated in 96-well microtiter plates for 48 hours. Immediately before use, the MTS and phenazine methosulphate solutions were combined, 20:1, and 20 µl was added to each well and allowed to incubate at 37°C for 4 hours. The conversion of MTS to formazan was stopped by addition of 25 µl of 10% lauryl sulfate, and formazan dye was quantified using an enzyme-linked immunosor-bent assay plate reader at 490 nm. Standard curves were determined to ensure correlation between cell number and absorbance. Proliferation curves were plotted as absorbance units ± SD compared with log drug concentration, and IC50 was estimated by fitting the data in points to a four-parameter nonlinear regression curve by using Prism (Version 2.0; GraphPad Software, San Diego, CA).[9]
To confirm the MTS results, cell count experiments were performed. The T98G glioma cells (2 x 105) were treated with OA at 0, 6.25, 12.5, 25, 50, 100, and 200 nM for 48 hours. The cells were trypsinized, centrifuged at 1500 rpm for 10 minutes, and resuspended in 100 µl of medium. After 20 µl was removed and mixed with 80 µl of Trypan blue (Gibco BRL, Grand Island, NY), 10 µl of the mixture was removed to undergo counting with a hemacytometer. Experiments were performed in duplicate and then repeated. Glioma cells (2 x 105) were pretreated with the caspase-1 and -3 inhibitors Ac-YVAD-aomk (250 µM) and DEVD-CHO (250 µM) for 2 hours before exposure to OA (0, 6.25,12.5, and 25 nM). Cell counts were performed as previously described. Probability values were determined by applying the Student t-test.
Apoptosis Assays
Cells were grown on 100-mm plates and treated with OA (25 nM) for 24 hours. Some cells were pretreated with caspase inhibitors as described previously. Treated cells were examined under light and fluorescent microscopy. First, the cells were washed in cold PBS, then 8 µl of 100-µM YO-PRO-1 dye and 4 µl of 1.5-mM propidium iodide was added for each 500 µl of PBS. After incubation on ice for 15 minutes, the cells were washed in cold PBS and observed under a fluorescent microscope. Live cells show little or no fluorescence whereas apoptotic cells show green fluorescence. Loss of cell integrity indicating necrosis results in red fluorescence.[10]
Immunoprecipitation and Kinase Assays
Following treatment with OA for various times, cells were harvested using a buffer containing 50 mM Tris-HCl (pH 8), 150 mM NaCl, 1% Triton X-100, 0.5% sodium deoxycholate, and supplemented with phosphatase and protease inhibitors: 5 mM sodium fluoride, 1 mM sodium orthovanadate, 4-(2-aminoethyl)-benzenesulfonyl fluoride hydrochlorine (ICN Biochemicals, Aurora, OH). After being lysed by repeated passages through an 18-gauge needle, the cell extracts were transferred to a microfuge tube and incubated on ice for 30 minutes. Cellular debris was removed from the soluble extracts by centrifugation at 12,000 G for 15 minutes at 4°C. The resulting lysates were precleared by incubation with protein A Sepharose (Pharmacia, Piscataway, NJ) for 1 hour at 4°C. After determining the protein concentration in each lysate, protein (50 µg) was incubated overnight on an orbital shaker at 4°C with 1 µg of anti-ERK, anti-JNK, or anti-p38 anti-body (Santa Cruz Biotechnology, Santa Cruz, CA). The immunocomplex was captured by adding protein A Sepharose (25 µl) to the protein mixture and incubating for 2 hours at 4°C. The beads were isolated by centrifugation at 2500 rpm for 5 minutes, followed by two washes in 1 ml of wash buffer (50 mM Tris-HCl [pH 7]) and one wash in 200 µl of kinase buffer (50 mM Tris-HCl [pH 7.4], 10 mM MgCl2, 1 mM dithiothreitol, and 1 mM sodium fluoride). The final pellet was resuspended in kinase buffer (30 µl) supplemented with 2 mM ethyleneglycotetraacetic acid, 10 µCi [32P] adenosine triphosphate (Amersham, Arlington Heights, IL), and the appropriate substrate: myelin basic protein (ERK1), GST-c-Jun (JNK), or GST-ATF-2 (p38) (10 µg) (Gibco BRL). Reactions were conducted for 25 minutes at 30°C to reduce background signal. The reaction was stopped by the addition of a 4 x concentrated sample buffer (10 µl). The samples were boiled for 5 minutes and 20 µl was loaded onto a precast sodium dodecyl sulfate-10% Tris-Glycine gel (Novex, San Diego, CA). After size fractionation, protein was transferred onto polyvinylidene difluoride membrane by using the Mini-Cell II Blot Module (Novex). The phosphorylated substrate was visualized by autoradiography.
Western Blot Analysis
Cells were harvested as described previously. Prior to loading, the protein concentration of each cell lysate was determined using a protein assay kit (Bio-Rad, Hercules, CA) and then diluted to 1 mg/ml in PBS. Protein (10 µg) was boiled in sodium dodecyl sulfate-polyacrylamide gel electrophoresis 4 x concentrated sample buffer, size fractionated on a precast 10 to 20% Trisglycine gradient gel (Novex), and transferred onto polyvinylidene difluoride membrane by using a Mini-Cell II Blot Module (Novex). All membranes were blocked with 5% non-fat dry milk and then incubated with anti-Bax antibody (Santa Cruz Biotechnology). Peroxidase-conjugated goat anti-mouse (Calbiochem) was diluted (1:1000) prior to addition. Each incubation was conducted at room temperature for 1 hour and was followed by a 15-minute wash (10 mM Tris-HCl [pH 7.5]), 100 mM NaCl, and 0.1% Tween 20). Detection of bands was performed using the ECL system (Amer-sham).
Electrophoretic Mobility Shift Assay
The NFB consensus oligonucleotide (Santa Cruz Bio-technology) was en labeled with [32P] adenosine triphosphate and T4 polynucleotide kinase (New England Biolabs, Beverly, MA) and purified by G-50 Sephadex columns (Pharmacia). Whole-cell protein extracts (25 mg) from cells treated with OA (20 nM) for 36 hours were added to 32P-NF oligo (~20,000 cpm, 0.2 ng) in a buffer containing 2 mg poly(dI-dC) (Pharmacia), 10 mg BSA, 10 mM Tris-HCl (pH 7.5), 50 mM NaCl, 1 mM dithiothreitol, 1 mM ethyleneglycotetraacetic acid, and 5% glycerol (total volume 40 ml). The DNA complexes were resolved on a 4% nondenaturing polyacrylamide gel containing 50 mM Tris, 0.38 M glycine, and 2 mM ethyleneglycotetraacetic acid, and run at 100 V for 3 hours at room temperature. The same procedure was followed for the super-shift assay except 2 ml of NFB p65 (A)X antibody (Santa Cruz Biotechnology) was incubated with the whole-cell extract for 30 minutes at room temperature prior to adding the 32P-NF oligo and reaction buffer.
Results
Okadaic Acid Induced Apoptotic Cell Death
The T98G, U87, and A172 glioma cells were grown in 96-well microtiter plates and exposed to increasing concentrations of OA for 48 hours. Using the MTS assay, we found inhibition of proliferation in all three cell lines (IC50 20-25 nM) (Fig. 1). To confirm the MTS results, we performed cell counts on OA-treated cells. Cell death increased with OA dose and similar IC50s were obtained for the three cell lines (IC50 20-40 nM) (Fig. 2). Treatment of T98G cells with caspase-1 and -3 inhibitors increased cell survival but did not prevent cell death (Fig. 3).
Figure 1. (click image to zoom) Graph demonstrating OA inhibition of proliferation of glioma cell lines. The A172, T98G, and U87 cells were grown in 96-well microtiter plates and exposed to OA for 48 hours. Using the MTS assay, absorbance at 490 nm was measured and plotted against log OA concentration (pM). The SD is shown for each data point (eight). A four-parameter curve was fit to the data points and used to determine the IC50.
Figure 2. (click image to zoom) Graphs showing the effect of OA on glioma cell proliferation (T98G [upper], U87 [center], and A172 [lower]) as determined by cell counts. Cells were treated with OA for 48 hours and then counted on a hemacytometer by using Trypan blue exclusion. Viability is expressed as a percentage of control (untreated) cells. The SD is shown for each data point.
Figure 3. (click image to zoom) Bar graph demonstrating the effect of caspase inhibitors on OA-induced cell death. The T98G cells were exposed to OA for 48 hours in the presence of caspase-1 and -3 inhibitors. Cell counts were performed and expressed as a percentage of control (untreated) cells. The SDs and results of the Student t-test are shown.
Subconfluent cultures of logarithmically growing T98G cells were treated with OA (25 nM) for 24 hours and examined microscopically. The cells underwent apoptosis as indicated by a rounded, but intact cell appearance, condensed nuclear chromatin, and blebbing of the plasma membrane (Fig. 4 upper left and lower left). Fluorescent staining with YO-PRO confirmed apoptosis as evidenced by green fluorescence (Fig. 4 upper right and lower right). Some treated cells exhibited red fluorescence, indicating that some necrosis occurred as well (Fig. 4D).
Figure 4. (click image to zoom) Photographs demonstrating apoptosis by YO-PRO/propidium iodide staining. The T98G cells were treated with OA for 24 hours and then stained with YO-PRO and propidium iodide. Upper Left: Untreated cells seen under phase-contrast microscopy. Upper Right: Untreated cells under fluorescent microscopy (same field as upper left). Lower Left: The OA-treated cells (phase contrast). Lower Right: The OA-treated cells under fluorescent microscopy (same field as lower left). Green staining indicates apoptosis, and red indicates apoptosis. Original magnification x 16.
Okadaic Acid-Activates Protein Kinase Signal Transduction
To determine the role of ERK, JNK, or p38 in OA-induced apoptosis we performed kinase assays on OA-treated T98G cells. Okadaic acid (20 nM) induced an increase in ERK activity that peaked at 12 hours and then declined (Fig. 5A). The JNK activity increased after 3 hours and was sustained at high levels for 48 hours (Fig. 5B). The p38 kinase activity was much less affected than MAPK or JNK (Fig. 5C).
Figure 5. (click image to zoom) Effect of OA on MAPK module kinases. The T98G cells were exposed to 20-nM of OA for the time points indicated and then underwent protein extraction. An in vitro kinase assay was performed on immunoprecipitated protein and then resolved by gel electrophoresis. A: The ERK with myelin basic protein as substrate. B: The JNK with GST-c-Jun as substrate. C: The p38 with GST-ATF-2 as substrate. h = hours.
Okadaic Acid-Induced IB Phosphorylation and NFB Transduction
To study phosphorylation of IB, we used Western blot analysis with an antibody specific to the phosphorylated form of IB. An increase in phosphorylated IB levels was noted after 6 hours of OA (20 nM) treatment. The levels peaked at between 12 and 24 hours then quickly decreased, indicating IB degradation (Fig. 6). Because IB sequesters NF B, OA-induced degradation of IB should lead to an increase in NFB binding. To test this, we performed an EMSA involving whole-cell protein extract and an oligonucleotide that contained the NF B consensus site. Okadaic acid caused an increase in NFB binding that was seen at 36 hours (Fig. 7). A supershifted band was shown in OA-treated cells when anti-NFB antibody was added to EMSA, thus confirming specificity of NFB binding (Fig. 7).
Figure 6. (click image to zoom) Western blot analysis of IB following OA treatment. The T98G cells were treated as shown and protein extracted at time points indicated. An antibody specific for phospho-IB was used.
Figure 7. (click image to zoom) Effect of OA on NFB activation as determined by electrophoretic mobility shift assay. The T98G cells were treated with 20 nM of OA for 36 hours. Protein extracts were probed with radiolabeled NFB oligonucleotide and resolved on a denaturing gel. A: An anti-NFB antibody was added to the reaction mixture to produce a supershifted band as indicated by the arrowhead. B: The position binding to the NFB oligonucleotide is indicated by the arrowhead.
Increases in Bax During Okadaic Acid-Induced Apoptosis
Overexpression of the proapoptotic protein Bax induces apoptosis by inhibiting the activity of Bcl-2. We used Western blot analysis to determine protein levels of Bax in cells undergoing OA-induced apoptosis. In T98G cells, OA (20 nM) increased Bax by 12 hours and peaked at 48 hours (Fig. 8). This late rise correlated with the time course observed in OA-induced morphology changes and DNA fragmentation.
Figure 8. (click image to zoom) Effect of OA on Bax expression. The T98G cells were treated with 20 nM OA for the times indicated and then underwent Western blot analysis in which an anti-Bax antibody was used.
Discussion
The dual-signal theory of apoptosis was proposed to explain the role of the c-myc oncogene in apoptosis. Apoptosis and proliferation are believed to be competitive and simultaneous pathways that may have common cellular mediators.[15] The ultimate fate of an individual cell depends on the intracellular balance between these pathways. We hypothesized that regulation of these two competing pathways occurs in part through the protein phosphorylation cascades within a cell.[5] In this study we showed that 20 to 40 nM of OA, a potent inhibitor of serine/threonine PP1 and PP2A, causes cell death, primarily by apoptosis in T98G cells. Our findings in three glioma cell lines are consistent with previous observations on the effective OA concentration and the time course of DNA fragmentation in human breast carcinoma cells.[4,21]
The three major pathways that mediate stress and survival signals from the extracellular environment are characterized by their terminal kinase: ERK, JNK, or p38. Using an in vitro kinase assay, we demonstrated an increase in ERK and JNK activity in T98G cells exposed to OA. This result is compatible with those reported previously by investigators who correlated JNK activity with apoptosis.[1,24,25] Thymocyte apoptosis induced by VM26, a DNA-damaging agent, and radiation treatment has been correlated with increased JNK activity.[39] Both ERK and p38 have also been implicated in apoptotic signaling.[1,24,44] This is the first report, however, in which the sensitivity of these pathways to OA has been demonstrated in glioma cells. At present we can only correlate kinase activity with glioma apoptosis. Although directly observed in interleukin-3-induced apoptosis, further proof will require more direct manipulation of the ERK and JNK genes in glioma cells.[12]
Using Western blot analysis and EMSA, we have shown that OA increases IB phosphorylation and NFB binding. Accumulation of phosphorylated IB is first seen at 12 hours, followed by its degradation at 24 hours, and thus resulting in nuclear translocation of NFB. As expected, we observed a significant increase in NFB binding at 36 hours. Although the time frame for these events occurs after the activation of the MAPK, we have no proof that they directly phosphorylate IB. The authors of recent reports have indicated that NFB inhibits tumor necrosis factor-induced apoptosis.[3,27,40,43] It is possible that the IB responses are epiphenomenona or it may be a cellular protective response against OA.
The Bcl-2 family of proteins is located in the mitochondrial membrane and protects against apoptosis by blocking caspase activity.[41] The proapoptotic protein, Bax, heterodimerizes with Bcl-2, neutralizing its protective effect.[32,48] Using Western blot analysis we observed a rise in Bax levels beginning at 12 hours and peaking at 36 hours. These results confirm recent observations that human breast carcinoma cells undergoing OA-induced apoptosis show an increase in Bax immunostaining at 36 to 48 hours.[36] Phosphorylation and activation of caspases play an important role in the induction of apoptosis.[18,26] To define further the involvement of caspases in OA-induced apoptosis, we treated T98G cells with a caspase-1 and -3 inhibitor. Both caspase inhibitors improved cell survival following OA treatment but did not eliminate all cell death. Although caspases are recognized to be an important mediator of apoptosis, OA may also trigger cell death by necrosis through noncaspase pathways as seen in other systems.[19,22]
Conclusions
In this study we described a potential signaling pathway for OA-induced apoptosis in glioma cells. Critical proteins along this pathway include JNK, ERK, NFB, and Bax. Because our study has focused on a single cell line, we cannot generalize these results to all glioma cells. Further studies of these signaling pathways triggered by OA may provide new targets for brain tumor therapies.
Reprint Address
Address reprint requests to: Lawrence S. Chin, M.D., Department of Neurosurgery, 22 South Greene Street, Suite S-12-D, Baltimore, Maryland 21201.
Abbreviation Notes
EMSA = electrophoretic mobility shift assay; ERL = extracellular signal-regulated kinase; IC50 = 50% inhibiting concentration; IB = inhibitory-B; JNK = c-Jun-N-terminal kinase; MAPK = mitogen-activated protein kinase; MTS = 3-(4,5-dimethylthiazol-2-yl)-5-(3-carboxymethoxyphenyl) -2-(4-sulfophenyl)-2H-tetrazolium; NFB = nuclear factor-B; OA = okadaic acid; PBS = phenazine methosulphate; PP = protein phosphatase; SD = standard deviation.